ALBUQUERQUE, N.M. — A bang and a swirl of dust from detonating 9 pounds of plastic explosive in the desert signaled the beginning of tests that — thanks to advances in high-speed cameras, imaging techniques and computer modeling — will help Sandia National Laboratories researchers study fragmenting explosives in ways that weren’t possible before.
“The details matter,” said Mark Anderson, principal investigator on Sandia’s fragment tracking project, which began explosive experiments in May. “Explosives are very complex to understand and to use, and they continually keep us humble.”
Researchers want to know how pipe bombs and other improvised explosive devices come apart and how much destruction they cause to learn how to mitigate that damage. They’d like to create computer models of explosive phenomena for broader studies since it’s impossible to do experiments for every possible situation, said Phillip Reu, team lead for diagnostic development on the three-year study and a member of Sandia’s Diagnostics Science and Engineering department.
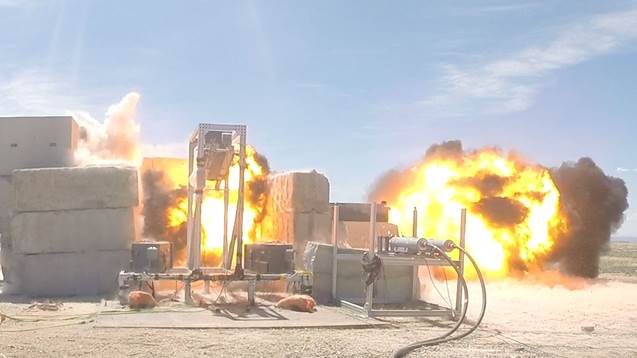
The project marries modern cameras, diagnostic technology and the latest computer algorithms to gather more data. Past techniques and equipment couldn’t provide detailed enough experimental data, forcing modelers to make assumptions about how materials failed or where fragments flew.
From a diagnostic standpoint, the dream is to be able to watch an exploding device expand, come apart and become fragments, then see the fragments fly and trace where they are in space and in which directions they go. That understanding could lead to better models and “what if” scenarios.
“We have to understand what creates the environment to mitigate the environment,” said Measurement Science and Engineering department researcher Tim Miller, who is developing algorithms to measure the shape and trajectory of explosive fragments.
Modelers, experimentalists exchange data
Modelers and experimentalists work in parallel. “The modelers give us a predicted outcome, which helps the experimentalists set up for the test,” said Anderson, a researcher in Sandia’s Energetic Systems Research department. “Then we take our measurements and feed them back to the modeler. The interchange is extremely powerful because we do better experiments having some modeling insights. And the modelers better see how their code works, and more importantly, where it doesn’t work, so they can unravel why that is.”
The improvements in diagnostic equipment allow researchers to better measure what goes on in an explosion. “We know that fragments travel at high speed, but we don’t really know the aerodynamic drag,” Anderson said. “When we see the size and shape of the fragment and we see it as it tumbles and rotates, we know the drag changes.”
Better instrumentation gives researchers new insights into such characteristics as mass, orientation, length-width ratio, velocity and tumble rate. “Is it one big chunk? Is it a bacon strip, is it a cornflake, is it a sausage link? Maybe it’s a steak coming at you,” said Engineering Sciences researcher Steve Attaway. “That’s the shape characterization.”
High-performance computing has improved the ability to calculate fracturing and fragments. “We’re running calculations now that 10 years ago we wouldn’t dream of attempting,” he said. “That said, every calculation is incomplete in what can be included, so the ability to gain more insight on how to make approximations to better mimic the fracture and fragmentation is important.”
How fast a fragment moves and whether it spins make a difference in the damage it inflicts. Simply assuming fragments are one size or behave one way skews the assessment of possible damage and ways to lessen it.
“If you think the average fragment is going to go a half mile, but you get one that’s shaped just so and it flies 2 miles, then that’s a problem, that begins to illustrate the range safety aspect of fragmenting explosive devices,” Attaway said. “We’re trying to get the best idea we can of that distribution.”
High-speed camera images aid sophisticated computer modeling
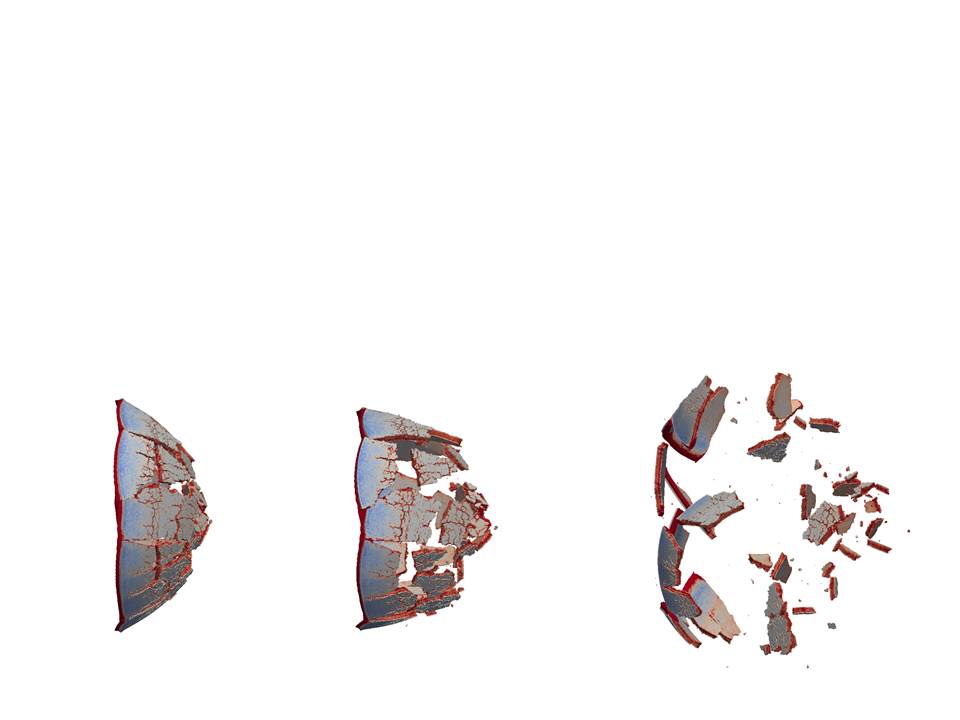
Today’s high-speed digital cameras take millions of frames per second, speeds that capture enough images to create measurements that feed complex computer models and help validate them. Sandia’s tests use two or more cameras to measure 3-D characteristics of fragments shooting through the air.
Cameras face a highly reflective background so fragments stand out. “These are things that happen very, very quickly so getting enough light and getting your cameras to go at the right time is very difficult to do,” Reu said.
The team also is working to develop high-speed X-ray movies of fragments. X-rays can create images of fragments in the initial stage of an explosion when smoke, flame and dust obscure what an optical lens can see. A high-speed X-ray movie would help tell the entire life story of a fragment, said X-ray and Non-destructive Evaluation department researcher Enrico Quintana.
While X-ray movies are not new, taking them at high speed is, he said. The team is developing a way to create continuous X-ray movies, rather than building up a series of images by firing nine Marx generators in rapid sequence as has been developed in the past five years. The team is slowly stepping up the speed, but it will be a giant leap to a movie that acquires hundreds or even thousands of images at rates of up to 1 million frames per second, Quintana said.
There are limits on the team’s high-speed camera and its X-ray scintillator, which converts X-ray photons to light photons. While the current setup could achieve nine images at a rate of 250,000 frames per second, the resolution wouldn’t be ideal.
“The important numbers to remember are the width of a single pulse from the flash X-ray system, 50 nanoseconds; the fastest shutter speed of the camera, 1 microsecond; and the primary decay time of the scintillator, 4 microseconds,” Quintana said. “Doing ultrahigh speed radiography is a matter of balancing the speeds of those three pieces of equipment, and we are limited by the slowest of the three.”
While evaluating new ways to protect the very expensive equipment during a detonation, the project successfully demonstrated X-ray movie capability. It provided sequential images from a multi-head X-ray system that captured the behavior of fragments in flight, beginning while the fragments were inside the explosive fireball and continuing as the fragments began to rotate in flight.
Lead technologist Mike Bejarano of the Measurement Science and Engineering department helps ready everything from cameras to cables and works on post-test data processing. The team is responsible for protecting the expensive tracking system and making sure it triggers when it should and that the delicate measuring system remains stable. “Moving even a tenth of a pixel introduces uncertainty and reduces the fidelity of the measurements,” Bejarano said.
Digital image correlation allows measurements of 3-D fields
Researchers use a technique called digital image correlation to watch how a metal case around an explosive expands and ruptures. They coat the object with a speckled pattern and set high-speed, high-resolution cameras in pairs for stereo photography. The cameras track how the pattern moves as the case explodes, and the stereo pairs show how speckles shift, allowing researchers to measure 3-D displacement fields and see when strains are great enough for metal to fracture. X-rays penetrate the smoke, flame and dust of the initial blast, capturing data cameras miss.
The project uses image-processing algorithm techniques developed by Diagnostics Science and Engineering researcher Dan Guildenbecher to help eliminate noise that interferes with accurate measurements.
Noise is defined broadly. “You’ve got things like shock waves, you’ve got dust that’s getting kicked up, you’re operating cameras at very fast rates and so they are inherently noisy. As a result, you’ve got noise that comes in with lots of different false signals that could show up in your measurement. We have a lot of different noise and we have to be able to figure out where we’re measuring actual particles,” Guildenbecher said.
Traditionally, explosive experiments placed layers of plywood or other material around a device to catch fragments — called soft catch — then removed the fragments for study. However, there was little or no diagnostics to evaluate fragments during flight. Access Delay and Structural Assessment researcher Jason Wilke said current experiments use high-speed photography and imaging techniques to determine fragment size and location during the explosion; researchers then dig the corresponding fragments from the soft catch for study.
Reu added, “What are you validating [the model] against, fragments that run into something or fragments that are floating in the air before they run into something? We’d like them in the air before they run into something because that’s how they’ll be coming at the things we care about.”
The team plans three to four test series a year, each with several explosions. “It’s better to run some tests, think about what went right, what went wrong, what we need to change, then come back in a few months and set up another test,” he said.