ALBUQUERQUE, N.M. — Sandia National Laboratories creates lightning in a lab to evaluate how anything from sensitive nuclear weapons components to entire buildings will hold up against the worst that nature might throw at them.
“Sandia’s primary mission is to assure an ‘always/never’ operating condition for nuclear weapons,” said Larry Schneider, senior manager of Sandia’s Electrical Sciences group. “They must work when authorized and never function otherwise. Direct strike lightning is a threat in the ‘never’ scenario.”
Researchers at the Lightning Simulator laboratory build upon the fundamental understanding of the physics involved in lightning to ensure that the incredible energy it releases cannot reach sensitive areas of a weapon, Schneider said. Their work includes developing complex theories and sophisticated computer modeling, “but ultimately, due to the complexity of the physics, it’s the testing that leads to confidence that the weapons remain safe even after a devastating event,” he said.
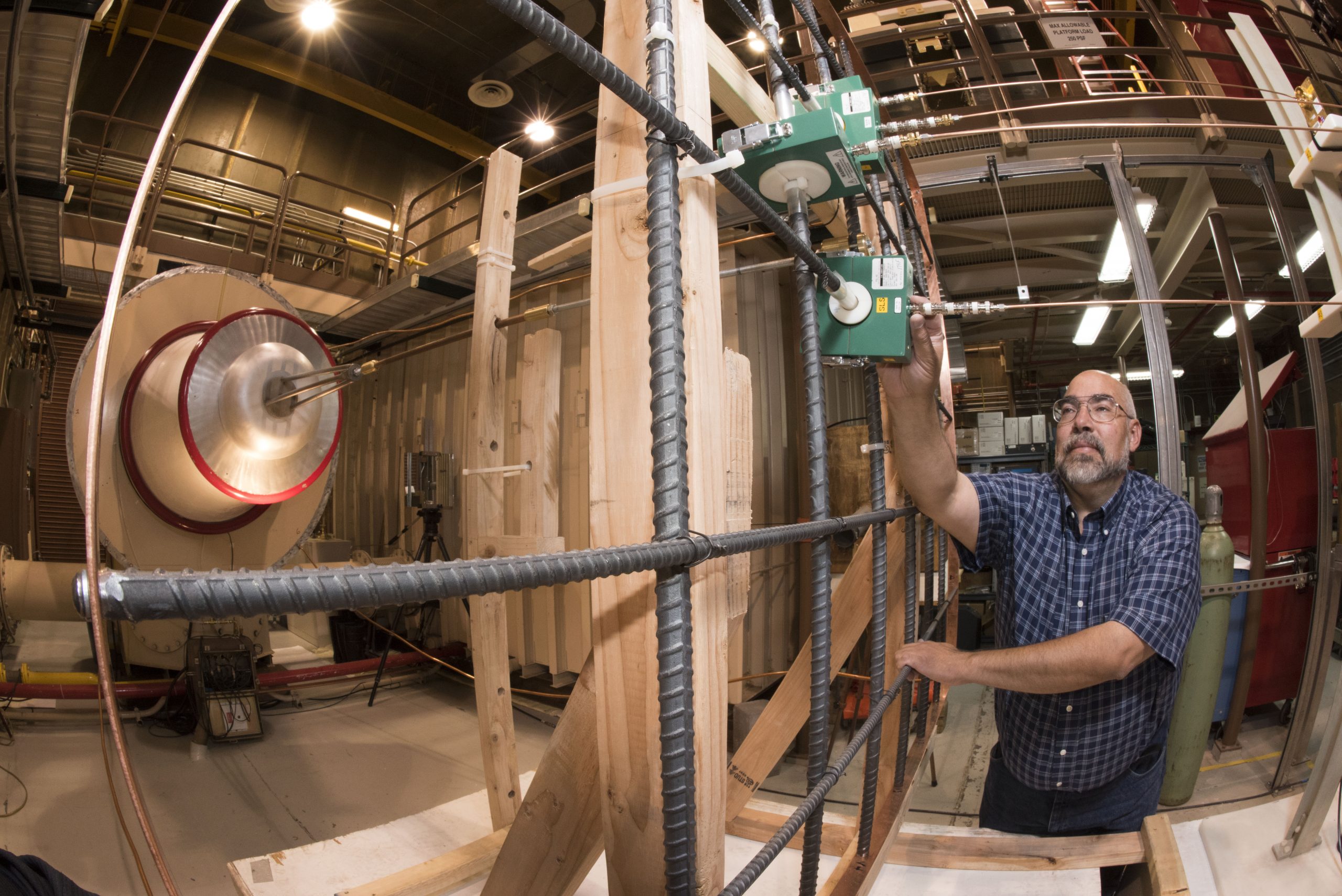
The lab’s electromagnetic qualification experiments support stockpile stewardship, helping ensure that small components, subsystems and nuclear weapon systems meet requirements whether lightning directly strikes the weapon or the missile or aircraft that carries it, or whether lightning strikes nearby and generates electromagnetic fields, said Leonard Martinez of Sandia’s Electrical Science & Experiments group.
Lightning Simulator experiments are part of a battery of tests Sandia conducts across a broad range of facilities to assure that stockpile nuclear weapons are safe, secure and effective. Electrical Science & Experiments manager Steve Glover said tests are a critical part of “validation and verification that the designs and manufacturing are correct and these systems are protected the way we intend.”
Sandia has performed lightning tests since the 1970s. Today, the focus is on improving understanding of the science behind lightning and the ways it can couple into systems, Martinez said.
Tests will help researchers understand lightning protection systems
For example, the Lightning Simulator is performing a series of tests to further researchers’ knowledge of lightning protection systems by looking at how lightning currents flow through rebar lattice structures and what electromagnetic forces are generated on lightning protection bond wire assemblies subjected to lightning currents of up to 200,000 amperes, Martinez said. The tests span the lab’s range of currents from 30,000 to 200,000 amperes. An ampere is a measurement of the flow of current in an electrical conductor.
Most of the tests are conducted on pre-fabricated samples of reinforced concrete walls, but researchers also measure currents at several locations in the lattice, without the surrounding concrete, to better understand current distribution in realistic configurations, Martinez said. They compare the measured current distribution to the results of their electromagnetic models to validate the models and conduct electromagnetic analysis to calculate expected forces on lightning protection bond wire assemblies, he said.
High-speed photometric cameras capture movements or deflections in the assemblies for additional analysis.
Experiments help guide creation of computer models
Experiments to understand the physics of lightning effects help Sandia’s Electromagnetic Theory group create better electrical and electromagnetic computer models to help predict how a nuclear weapon system will react if hit by lightning.
“We try to understand how much energy, in terms of voltage and current, penetrates a weapon due to direct strike lightning,” said the group’s Roy Jorgenson, a computer scientist specializing in electromagnetics.
Lightning Simulator experiments, in combination with smaller-scale experiments to validate models, guide the creation of analytical and empirical models, he said. The group uses a combination of analytical techniques and codes within a suite of codes called Ramses.
“The Lightning Simulator is the tie between the actual lightning event in nature and small-scale experiments,” Jorgenson said.
Lightning is an electrical current, like a massive jolt of static electricity. Thunderclouds have electrical charges — lighter, positively charged particles on top and heavier, negatively charged particles on the bottom. When a cloud’s positive and negative charges are large enough, they try to neutralize by reaching each other, causing a huge spark — lightning — between them. Most lightning occurs within clouds but negative charges also are attracted to positive charges on the ground, concentrated around taller objects such as trees. When those charges connect, lightning hits the ground.
The eye sees a single bolt, but a lightning flash consists of many return strokes, moving too fast to see separately. A cloud partially discharges its energy when the first stroke hits. The remaining energy continues to flow in residual current, also called continuing current, and may lead to a return stroke.
The lab creates a lightning pulse by discharging high-voltage capacitor banks known as Marx generators, housed in two high-voltage tanks filled with insulating oil. The simulator’s charge console controls when lightning pulses from the generators are released. Each generator puts out a return stroke, and a continuing current source can be injected in between pulses, much like natural lightning.
The simulator can generate strikes up to a maximum peak current of 200,000 amperes for a single stroke, 100,000 amperes for a subsequent stroke and several hundred amperes of continuing current for hundreds of milliseconds. In direct lightning tests, the simulator is connected to an object or arcs to it to simulate a lightning strike. In indirect tests, the lightning current is close by but doesn’t actually strike the object. This lets researchers study how magnetic fields generated by the nearby strike affect test objects.
Sending a signal to simulator to fire
Operators charge and fire the machine from a control room, sending a signal throughout the simulator to fire in sequence. Data flows into a screen room, so-called because its walls screen out electromagnetic waves that would interfere with diagnostic recorders. Fiber optic transmitters send information from the experiment into the screen room’s fiber optic receiver, where it’s converted into an analog signal that can be viewed on an oscilloscope for analysis.
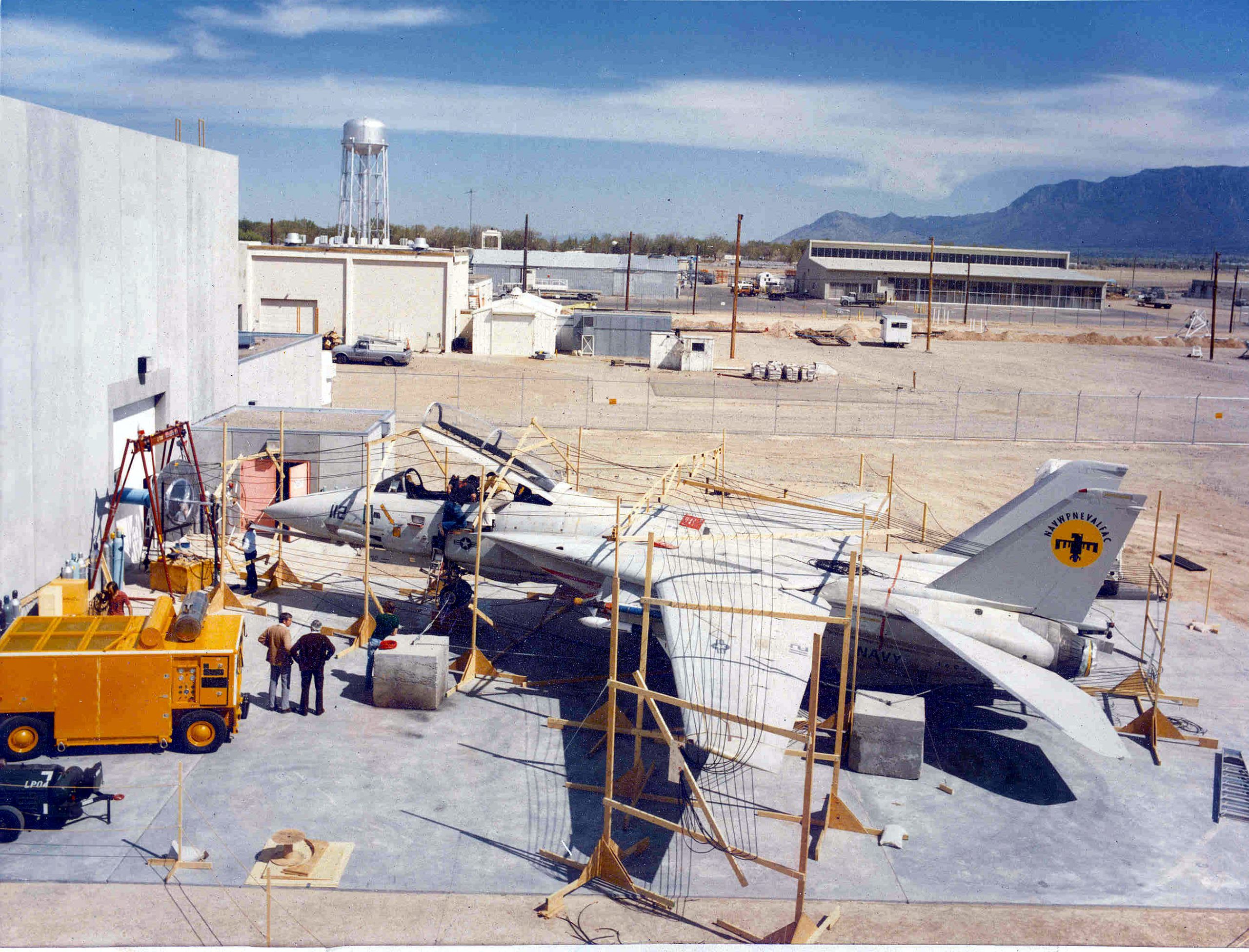
The lab does 200 to 300 tests a year, and operators can fire the machine several times a day, Martinez said.
Other agencies call on Sandia’s expertise in high-voltage science.
The Mine Safety and Health Administration (MSHA) asked for help in determining the cause of a Jan. 2, 2006, explosion in West Virginia‘s Sago Mine that killed 12 miners. MSHA asked Sandia to study whether energy from a lightning strike could travel underground to ignite an explosive mixture of methane gas trapped in a sealed section of the coal mine. A Sandia team spent 10 days at the site, and characterized a mechanism that accident investigators hadn’t previously considered — that current from a surface lightning strike can generate electromagnetic fields that spread through the earth. The findings were part of MSHA’s investigation report to Congress.
In 1982, the simulator did its largest test ever, for the Naval Air Systems Command, which wanted to know how a lightning strike would affect the sensitive computer electronics of an aircraft. The lab subjected an F-14A parked at the rear of the lab to an 80,000-ampere pulse that entered through the nose and exited via the wing tip. The charge, lasting less than a second, coursed through a series of cables back to the lab to be measured. The simulator building has since expanded into where the plane was parked, so such a test is no longer possible, Martinez said.